
The Hopter project
is being developed by the Space Research Centre of the Polish Academy of Sciences, the WROONA project, Astronika Sp. z o. o., and the Angström Space Technology Centre., and the Finnish Geospatial Research Institute.
See the presentation at the 11th Low-Cost Planetary Missions conference in Berlin (June 9-11, 2015).
The Hopter is an innovative locomotion system designed to investigate in situ planetary mountains and ultra-low gravity Solar System bodies.
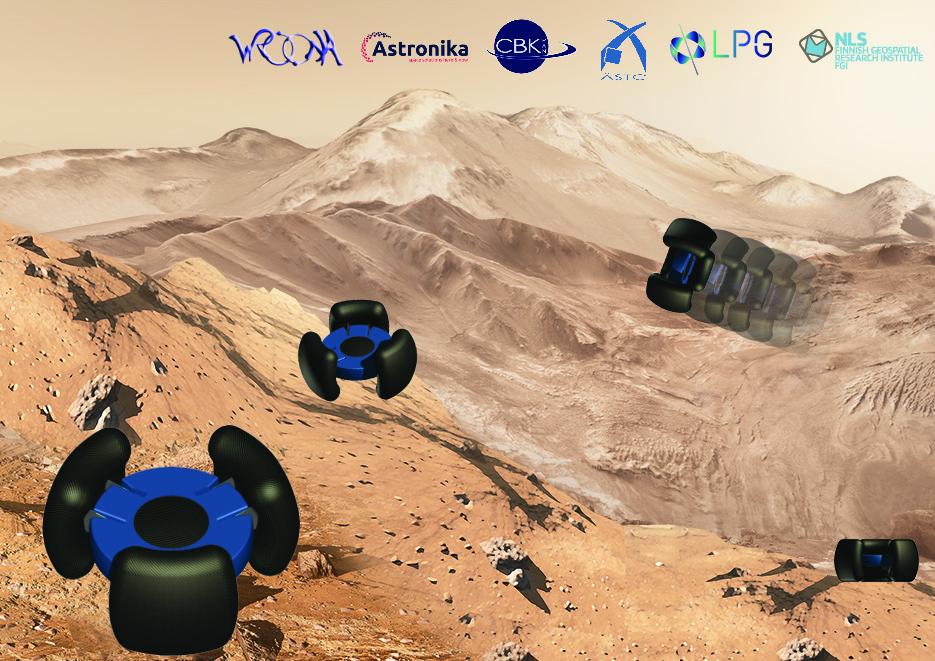
Planetary exploration: problems with the current mobile exploration platforms
Exploration of ultra-low Solar System bodies: lessons from the Philae platform
HOPTER: the Highland Terrain Hopper
Mopters, Phobters and Kbopters
The Hopter compared to previous space
hoppers
Planetary exploration:
problems with the current mobile exploration platforms
All the known solid bodies of the Solar System have gravity lower than the Earth. The platforms currently used for the exploration of these bodies include rovers and landers. Landers consist of an immobile platform from which analysis of the ground and environment is performed; their strength is therefore, in addition to accurate in situ observations, the ability to monitor events at that site, for instance weather patterns. The strength of rovers lies in the possibility of exploring many kilometres; however two severe restrictions apply: (1) related to landing and trafficability (both place dramatic constraints on slopes and terrain roughness throughout the 10s of km wide landing ellipse; e.g. [1]); (2) related to the surface gravity: roving relies on adherence of wheels to the ground, so that on ultra-low gravity bodies such as Phobos and Deimos, asteroids, cometary nuclei or Kuiper Belt Objects (KBOs), roving is not possible.
The landing and trafficability issues cause major problems for geology. Most of the knowledge of field geologists is learnt from highland terrains indeed, whether on land or in the sea, because mountains give a three-dimensional view of the geological processes. Roaming around provides information on their geographic extent as well as on their evolution through time (Figure 1). Escaping the landing ellipse takes a lot of time: although the dimensions of the landing ellipse for the MSL/Curiosity rover was smallest ever (20 x 25 km), it took the rover 692 Earth days to cross its landing ellipse border [2], meaning that:
(1) due to the nearly flat terrain constraints on planetary landing and roving, the view that rovers give us can be compared to the view that geologists would have of the Earth if only kilometres of regions such as the Sahara and the Mississippi delta were explored;
(2) the instruments and the main system are already 2 years old once the 'Sahara reg landing constraints' is released; the observed damages on the Curiosity wheels testifies to this concern (Figure 2).
People
team lead by:
Jerzy Grygorczuk, Chief Engineer
Daniel Mège, Chief Scientist
Core team members are:
Joanna Gurgurewicz, researcher
Łukasz Wiśniewski, engineer
Marek Banaszkiewicz, researcher
Hans Rickman, researcher
Downloads and links
Figure 1. One of the most astounding achievements of field geology: west-east geological profile across the Alps, an excerpt of an evolution scenario of the Alps, one of the most complex terrestrial orogens, proposed by Swiss geologist Emile Argand in 1916. The modern geological and geophysical works have remarkably confirmed this interpretation. It is especially consistent with plate tectonics, which was theorised 51 years later.
![]() |
Figure 2. Damaged Curiosity wheel in August 2014, after roving on flat terrains during 2 terrestrial years. |
The lifetime estimate of the Curiosity wheels on the terrains it was investigating in 2014 corresponds to 8-14 km, which motivated revision of the planned route to Mount Sharp, its destination [3]. The question of accessing interesting terrains beyond the landing ellipse has been recognized critical [4]. Surfaces of loose materials such as debris slopes of crater walls also prevent from accessing overlying crater wall outcrops.
The adherence issue for ultra-low gravity objects limits exploration of these objects to landers, i.e. to a study of a single site at the surface, chosen as a function of the landing constraints (slopes, rock abundance, spacecraft motion relative to the studied object…) and instrument requirements (communication with the orbiting spacecraft or the Earth, sunlight…), not significantly of the intrinsic scientific interest of the landing site compared to other potential sites.
Mandatory is to access planetary mountains in order to gain 3-dimensional views of the surfaces, as well as having mobility within these mountains. On Mars, such mountains are, for instance, Valles Marineris, Cerberus Rupes, Olympus Mons basal scarps and slopes. On the Moon, steep rille walls display crustal outcrops, and impact basin rims and central peaks open a window to the materials that compose the deep lunar crust.
Exploration of ultra-low bodies: lessons from the Philae platform
After release from the Rosetta spacecraft, the Philae lander bounced twice before being ar-rested against a rock, in spite of a sophisticated mechanism that should have kept it at the site of first touchdown. The MUPUS instrument on Philae, designed and built at the Space Research Centre (SRC) PAS in Warsaw (under contract with DLR), worked perfectly well and returned scientific data that are currently being analysed.
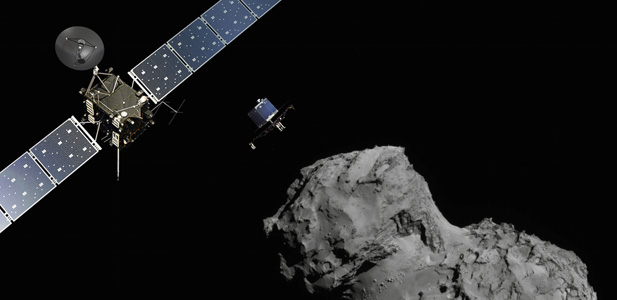
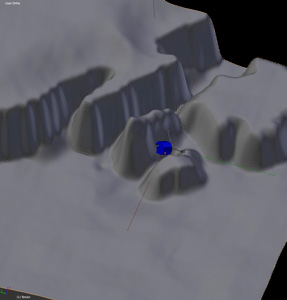
Figure 3. Philae release from
Rosetta (credit: ESA/Rosetta/Navcam) and modelling of Philae
orientation after its final rebound. Philae is blocked by the
surrounding topography (credit: ESA/Rosetta/Philae/CIVA).
This calls for the following remarks:
1. Predicting a final landing site on a very low gravity body such as a cometary nucleus or an asteroid is extremely difficult (and expensive);
2. Why not take advantage of bouncing instead of attempt to avoid it? The Philae jumps illustrate that jumping on low gravity bodies is easier and more efficient than any other locomotion system and allows, with a very small quantity of energy, to investigate large portions, or the whole surface of the studied bodies in detail. Jump analysis is also able to provide information on the subsurface structure ot the studied body.
3. With MUPUS, SRC PAS has demonstrated skills to develop original, complex and efficient low gravity space systems. This offers a huge potential for future space exploration. The importance of landing in low gravity conditions is critical to space exploration today indeed, for scientific research and investigation of resources, as most solid bodies in the Solar System have a gravity field which is lower than the Earth's.
Lessons from the Moon and other neighbours
Video
1. Apollo 17 astronaut Eugene Cernan jumping and
discussing with Harrison Schmitt: jumping is the best
way of moving on low-gravity bodies. 167:09:45 Cernan: (Doing long, two-footed hops) This is the best way for me to travel. Uphill or downhill. 167:09:48 Schmitt: What's that? 167:09:50 Cernan: Like this. Two-legged hop. 167:09:53 Schmitt: There seems...Yeah. 167:09:54 Cernan: And on level ground, I can skip. I don't like that loping thing. 167:09:59 Schmitt: Oh, the loping's the only way to go. 167:10:01 Cernan: Well...See, when I'm on level ground, I can skip. But this two-legged thing is great! Man, I can cover ground like a kangaroo! Transcript is © 1995 by Eric M. Jones. |
Jumping is also the main locomotion system for a number of animal species. Galagoes (Video 2) are among these, and the Highland Terrain Hopper has been nicknamed Galago.
Video 2. Jumping animals: galago and others, © BBC.
HOPTER: the Highland Terrain Hopper
Project's vision:
HOPTER:
The HOPTER project, supported by 15 planetary science laboratories in Europe and the USA, is a Polish project developed by the team who built the MUPUS instrument on Philae, led by Dr Grygorczuk, with contribution of a planetary geosciences team based at the Institute of Geological Sciences PAS in Wrocław and in connection with the nascent Polish space industry (Astronika Sp. z o. o.).
The project aims at developing HOPTERS: all-terrain, efficient, science focused, but low cost hoppers to study the surface of low gravity bodies, from the Moon and Mars to much smaller objects such as asteroids, cometary nuclei, and natural satellites.
The HOPTER is a unique locomotion system that not only allows to work on very low gravity bodies, but also can accurately jump across topographic obstacles the size of which is inversely proportional to gravity. For instance, a hopter can jump up to 4 m high on Mars (figure on the left), 9 m on the Moon, and to any height above a cometary nuclei provided that the jump speed does not exceed the escape velocity. Actually, this new locomotion system is a whole family that includes 3 main members, depending on the surface conditions of the desired studied object.
Mopters, Phopters and Kbopters
Hopters (Fig. 3) are divided into three groups (Figure 5).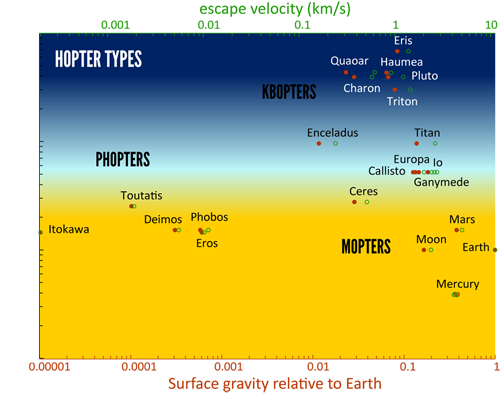
Figure 4. The HOPTER family
• Kbopters are hopters that are adapted to the ultra-low temperatures (typically 30-40K) that are encountered at the surface of KBOs, and cometary nuclei at similar distances to the Sun.
Hopter features
A hopter with a miniaturised payload will keep within 10 kg for a diameter of 50 cm (Figure 5).
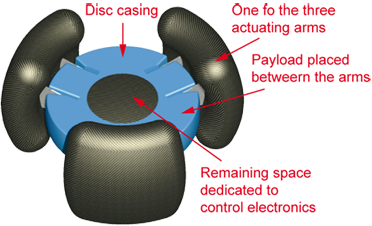
(1) It makes the hopter much more affordable than other exploration platforms. For comparison, the mass of MSL/Curiosity is 899 kg;
(2) Unstable slopes, like impact crater slopes, are not accessible to rovers but can be accessed by hopters;
(3) It allows to consider sending several hopters in the same mission, opening the potential of networking in the field, as geophysicists do during e.g. seismic surveying (Figure 6, right);
(4) It makes risky scientific objectives achievable. The loss of one inexpensive platform while realising an ambitious scientific objective within an inexpensive 10-hopter mission may not endanger the mission.
The currently investigated displacement strategies are illustrated on Figure 6.
Figure 6. Inventory of hopter displacement strategies
Current development stage
The Hopter compared to previous space hoppers
The first part of this section is a contribution from Łukasz Wiśniewski.
Usage of reaction wheels is enough to perform gentle movements on the surface of low-gravity bodies, but insufficient on bodies with higher gravity field (e.g., Moon, Mars). The large Mars Reconnaissance Lander (~500 kg; Figure 7e) uses a radioisotope stored in thermal rocket engine and repeatedly compresses CO2 and discharges it through thrusters [11]. It takes advantage of performing short, controlled ballistic flights to achieve distances of c.a. 1.5 km, giving the capability to cross terrain inaccessible to conventional rovers, but in fact still cannot access those since it still requires flat terrain for landing. The 1.3 kg Elastic Cage Design (Figure 7f) protects the payload and mechanism by multiple bended metal strips arranged as ribs. Jumping is achieved through cage compression and sudden release along the vertical axis [12]. The partially prototyped design of hopper with Shape Memory Alloy (SMA) Actuator (Figure 7g) is tetragon-shaped; after each jump it flips back to the starting position. The SMA actuator is driven by the day/night temperature gradient and, since only one actuator is proposed, the angle of jump is fixed [13].
The hopter combines advantages of some of the hoppers on Fig. 3, but more importantly, adds key features that are fundamental for a wide range of scientific applications:
- Simplicity, which increases reliability and robustness;
- Ability at implementing a variety of displacement strategies;
- Versatility: hopters may be considered as main mission platforms, secondary platforms connected to a lander or rover for exploring risky terrain, or a help to human in situ exploration in areas where the scientific reward is worth the potential loss of a hopter but not a human being.
- High manoeuvrability: the symmetric distribution of three actuating legs determines the jumping direction by adjusting jump energy in each arm separately. Hopters are also symmetric (no top, no bottom), which in case of unpredicted jump target behaviour ensures mobility recovery;
- Extreme mobility: jumping high is made possible by the low mass and small size; obstacles as high as 1.5 m on Earth, 4 m on Mars and 9 m on the Moon can be overcome, which in most circumstances on Mars and the Moon is enough to travel far in mountains. Much higher cliffs can be crossed if ~1x1 m benches divide the cliff into portions having subvertical walls lower than these values. The battery autonomy objectives correspond to 1 000 jumps/hopper without recharge. It will shown in part B2 that the comparative locomotion efficiency of a hopter on flat terrains is ~70 times higher, in terms of distance/kg/day, than the Curiosity rover (the same comparison for rugged terrains being irrelevant).